Advances in Research
Over the past few years, the scope of basic and translational research has flourished under the leadership of Stavros Thomopoulos, PhD, Director, Carroll Laboratories for Orthopedic Surgery, and Vice Chair, Basic Science Research, Department of Orthopedic Surgery. This has included the recruitment of both PhD and clinician-scientists whose work is related to orthopedic care. Joining these new faculty were members of their research labs, resulting in nearly 30 researchers, technicians, and trainees now involved in the Carroll Labs alone.
“The Carroll Labs supports basic and translational research in orthopedics, which is critical for driving innovative and transformative changes in patient care down the road,” says Dr. Thomopoulos. “The expansion of our research team, integration of our research endeavors, and collaborations with orthopedic surgeons, bioengineers, and other specialists are generating exciting and productive developments for therapeutic applications.”
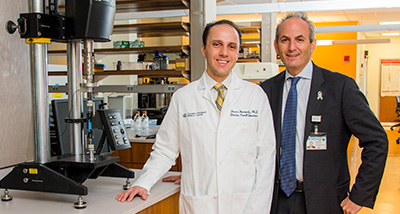
Dr. Stavros Thomopoulos and Dr. William N. Levine
In addition to recruitment, the Carroll Labs hosts a monthly seminar series presented by leaders across the country in the field of musculoskeletal research. The invited lecturers also meet with relevant faculty throughout the day as well as with residents and fellows. “This has been a programmatic advance that allows us to expand the scope and quality of our interactions with the broader community,” adds Dr. Thomopoulos. “The series has been very well received on campus, particularly with engineers who are interested in musculoskeletal questions. It’s a good opportunity to exchange ideas and gain some insight and advice as to what we do. And it’s led to some collaborations as well.”
Thriving Research Endeavors
Tackling Intervertebral Discs
Nadeen O. Chahine, PhD, Associate Professor of Bioengineering in Orthopedic Surgery and Biomedical Engineering, joined the Department of Orthopedic Surgery in 2017. Dr. Chahine’s research lab focuses on both degeneration and regeneration of the intervertebral disc, applying tools of bioengineering, cell and tissue mechanobiology, and animal physiology to study the function of the disc and disc cells.
Her team is currently collaborating with the Departments of Orthopedic Surgery and Rehabilitation Medicine investigating potential systemic biomarkers of intervertebral disc disease severity and back pain. Over 200 clinical subjects have been enrolled and preliminary findings indicate that back pain patients have elevated systemic inflammation in a manner that depends on the diagnosis of the disc pathology. These findings confirm their hypothesis that inflammation is a driving force of pain propagation associated with spinal degeneration. They have identified a profile of mediators that are correlated with improvement in pain and disability with epidural steroid injection treatments. Their work suggests that identifying markers to accurately predict response to treatment may become an additional diagnostic tool to spine radiological examinations.
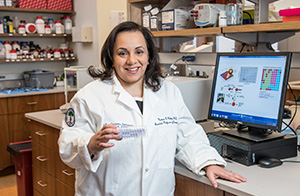
Dr. Nadeen Chahine and her lab team are developing novel 3D printed scaffolds with optimized functional biomechanical properties for loadbearing applications.
Another area of interest of Dr. Chahine’s lab is understanding the causes of degeneration, with a particular focus on adjacent segment degeneration, a complication following fusion. To address this, Dr. Chahine is investigating treatment paradigms that can maintain some physiological level of mechanical loading in a repaired disc. Using newer technologies, including 3D printing, she and her team are seeking to construct scaffolds and materials that are more in line with the native mechanical properties of the human disc. They have been evaluating a novel polymer biomaterial and using a formulation that makes it viscoelastic, a rubber-like material that exhibits the mechanical behavior of biological tissues. By taking different polymers and optimizing the conditions in which the researchers can print them in a 3D configuration, they can reproduce the mechanical properties that represent the nucleus pulposus and the annulus fibrosus. The researchers will now test this tissue in an animal model or through in vivo studies to evaluate the responses to implanting it in the body.
Improving Treatments for Cerebral Palsy
Jason B. Carmel, MD, PhD, joined Columbia in 2018 as the Director of the Movement Recovery Laboratory, and Scientific Director of the Weinberg Family Cerebral Palsy Center. A child neurologist and motor system neuroscientist interested in central nervous system injury and repair, Dr. Carmel pursues research to restore movement after paralyzing injury. He and his team study how plasticity of the motor systems changes with age and how developmental plasticity can be leveraged to promote recovery after injury. His laboratory uses activity-based repair strategies, including brain and spinal cord stimulation, to strengthen their connections. The animal models of disease they employ are used to improve the understanding of injury and ultimately improve movement in people with brain and spinal cord injury.
The Weinberg Center conducts research to understand the etiology of cerebral palsy. An emerging area of research is in the genetic causes of the disorder. The Weinberg Center collaborates with the Institute for Genomic Medicine to try to determine the genetic contribution, and they are part of the International Cerebral Palsy Genomics Consortium. In addition, the Center seeks to define the natural history of CP. The clinical experience of the team suggests that function is lost sooner in people with CP than in typical aging, and the studies will track function over time to determine the degree to which this observation generalizes.
The Center's research also seeks to restore movement through advances in orthopedic care, robotic therapy, and physical rehabilitation. Each intervention is tested independently to understand whether it contributes to better walking, sitting, or arm and hand movements. In addition, the Center is ideally situated to test the efficacy of various interventions to improve movement head-to-head. Clinicians from orthopedic surgery, rehabilitation medicine, and physical therapy, along with robotic engineers approach the restoration of movement very differently. With members of each of these disciplines, represented in the Center, the researchers can test their approaches against one another for the first time.
Physiological Mapping and Photothrombotic Lesion of Internal Capsule
IC Mapping
Intracortical sharp micro-electrodes were used to electrically stimulate the internal capsule region of the subcortical areas. Forelimb area is represented in green and hindlimb areas in red bubbles. The size of the bubbles are proportional to the excitability of the areas mapped.
Photothrombotic Lesion
The hotspot identified by mapping the forelimb area using the IC mapping is photothrombotically lesioned. A brain section with the lesion is shown with (A) TTC staining and (B) cresyl violet staining. Arrows are pointed at the site of lesion.
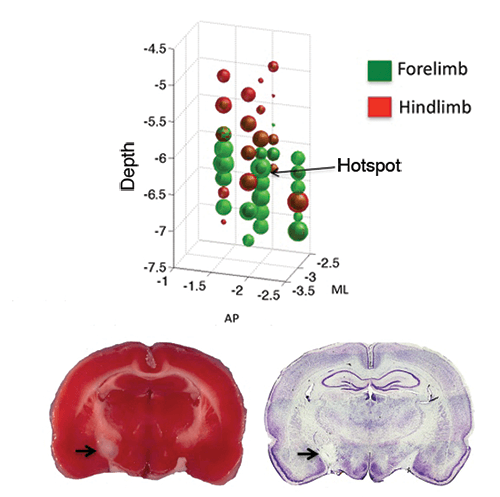
A Surgeon with a Basic Science Focus
David Kovacevic, MD, joined Columbia in 2018 as Chief of the new Shoulder, Elbow, and Sports Medicine Service at NewYork-Presbyterian Lawrence Hospital. Dr. Kovacevic holds a K08 clinician-scientist research career development award from the NIH to investigate inflammation in rotator cuff tear and repair. He also co-leads the American Shoulder and Elbow Surgeons Massive Rotator Cuff Tear Research Group, an international collaboration, which seeks to improve the understanding, treatment, and management of massive rotator cuff tears. Dr. Kovacevic’s own research resides in the crossover realm between laboratory and animal research with an eye on applications to clinical care. He is currently studying genes and proteins that regulate bone-tendon healing, using this knowledge to develop better biologic treatments for tendon injuries and prevention of muscle degeneration.
Tendon-to-Bone Attachment on Land and in the Sea
Dr. Thomopoulos’ lab is focused on a number of very challenging clinical scenarios for surgeons, which is essentially any scenario where a soft tissue has to be connected to a hard tissue; for example, tendon to bone, meniscus to bone, and ligament to bone. Because these connective tissues are “ropelike” in their structure and mechanics, connecting them back into a very rigid and cement-like material presents challenges for which the solutions are not obvious. “The current technique of driving suture anchors through the tendon and into the bone is like driving a nail through the rope and into the cement,” notes Dr. Thomopoulos. “But the soft tissue doesn’t integrate into bone well, so the approach is not very effective mechanically, and failure rates are still quite high.”
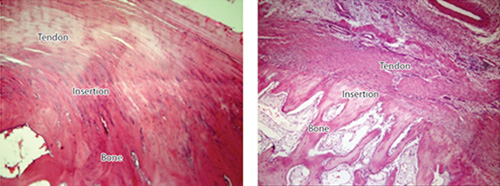
The functionally graded transition between tendon and bone is not regenerated during the healing process. (Left) Healthy attachment (Right) Healing attachment
Dr. Thomopoulos breaks down the challenge into two issues — the initial repair strength and the healing process — and is working to develop solutions to both. In recent years, the researchers have been making progress with tissueengineered solutions that promote the right healing response — whether with growth factors or scaffolds with cells, for example. These studies continue, with progress being made in identifying a specialized stem cell population specific to the attachment site that builds the interface during development. “Now that we’re able to purify this population, we want to determine its therapeutic potential for repairing the tendon-to-bone attachment,” says Dr. Thomopoulos. “While the research is still in the early stages, we are excited about the promise this cell population holds because it is unique to this site.”
Columbia faculty are studying genes and proteins that regulate bone-tendon healing to develop better biologic treatments for tendon injuries and prevention of muscle degeneration.
The researchers have also moved forward with translational studies involving scaffold designs in larger animal models developed in collaboration with colleagues who are expert in materials science. “If we can show good efficacy in these models, we can then pose a pathway to the FDA to move us closer to patient trials,” adds Dr. Thomopoulos.
Mussels and barnacles must be doing something right and, with that in mind, Dr. Thomopoulos and his colleagues are seeing if they can replicate the way that various marine organisms have figured out how to attach themselves to rock surfaces. “Literature is available that has given us a better understanding of how these organisms are able to create this incredibly strong adhesive connection to a surface in an underwater environment,” he says.
Collaborating with a professor in polymer chemistry, they are developing polymerbased versions of mussel biochemistry to create an underwater adhesive. To that end, they have created films of these polymers in a very specific range of mechanical properties that can improve the repair and will work in the arthroscopic underwater environment. “It’s like putting Krazy Glue between the tendon and bone, but under water,” says Dr. Thomopoulos. “While it is based on the chemistry of mussel adhesion, it also has a very specific set of mechanical criteria.”
While they have successfully completed proof-of-concept experiments using various glues, they are still searching for the right combination of polymers based on this mussel biochemistry.
Another novel approach, also in the early stages, involves modeling and proofof- concept experiments inspired by the geometry of python teeth. “One of the really interesting evolutionary consequences of tooth shape is that the geometry of teeth in various animals is actually driven by the functional requirements of that animal,” explains Dr. Thomopoulos. “For example, the python’s teeth are designed for grasping. A python essentially dislocates its jaw and wraps its head and mouth around the prey and then sinks those curved teeth into the flesh of the prey. The specific curvature, sharpness, and the conical shape of the teeth are optimized to go into soft tissues, such as muscles or tendons, and prevent them from slipping out.”
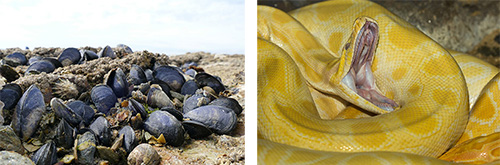
Dr. Stavros Thomopoulos is exploring novel concepts beyond the bench in the biochemistry of mussels and the geometry of python teeth to address the implacable challenges of tendon-tobone attachment.
“The idea that we’re trying to implement is for a grasping device for tendon-to-bone repair,” continues Dr. Thomopoulos. “If you imagine an array of python teeth between the tendon and bone, you essentially have the same concept where the tendon is trapped into the teeth. In the clinical setting after a tendon-tobone repair, as the muscle pulls on the repaired tendon, the tendon could retract and possibly rerupture, but these teeth would just trap the tendon more. Our experiments and models have defined the stress fields and grasping capabilities of different tooth geometries. Using 3D prints of different tooth geometries, we tested how well they could grasp a tendon in the lab. The idea of both the mussel-inspired glue and the python-inspired teeth is to spread the forces across the entire surface area of the repair, distributing the forces and reducing the stress concentrations. If we can keep these two tissues up against each other after surgical repair, there’s a much higher chance for a positive longer-term healing outcome.”